3.4. Map and pathways of the energy transition
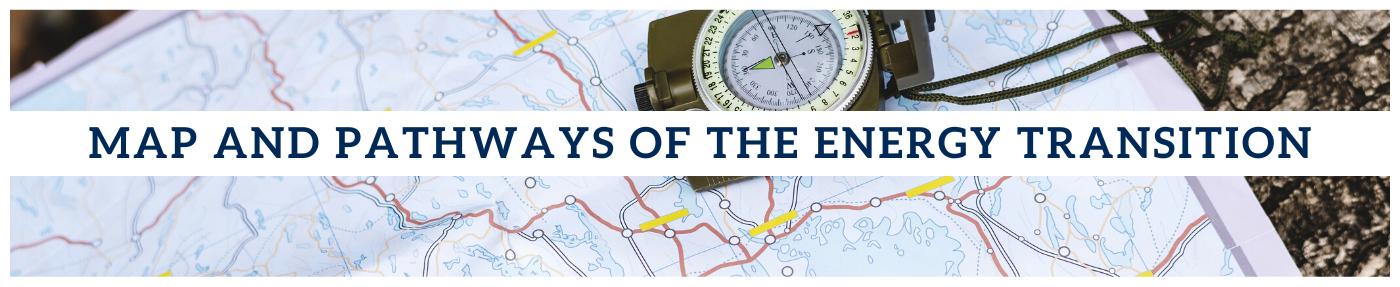
3.4 Map and pathways of the energy transition
British mining giant Anglo American is planning to mine a multi-metal deposit beneath the Viiankiaapa Mire Reserve in Sodankylä. The mine would produce, for example, copper and nickel which are used in batteries and many electrical appliances. Conservationists and locals have fought against the mining project, as it could damage the mire ecosystem at Viiankiaapa (Photo: TuomoS, Wikimedia Commons, CC BY-SA 4.0.)
In this section, we look at how energy systems should be changed to achieve a sustainability transition. It has been long argued in research literature that the barriers of energy transition are not primarily technological or economic, but political and cultural. Today, this idea has become mainstream, especially as wind and solar power have already become the cheapest new forms of electricity generation in most parts of the world.
Climate change is probably the main driver of the energy transition, but the issue of energy security has also been central to energy systems throughout history. Its importance has been heightened by Russia's military action in Ukraine in the 2020s. Russia's war of aggression has shown how vulnerable critically important systems, such as food and energy systems, actually are. The causes of the war are disputed, but some scholars have suggested that the underlying cause is the struggle for energy resources and their markets. According to this view, Russia's military action is aimed at preventing the strategically important Black Sea gas and coal deposits in eastern Ukraine from slipping out of Russian control as Ukraine's engagement with the European Union intensifies. This would be a resource war linked to fossil fuels; similar resource wars have happened in human history repeatedly.
An energy transition to renewables will require huge investments, but at best it can also lead to a more inclusive and equitable global economy, as well as a more climate-sustainable one. At the same time, the geopolitical landscape around energy is changing – most likely making the world less prone to crises.
The energy transition is underway – but the pace is not fast enough
The rapid growth of wind and solar power over the last couple of decades shows that the energy transition has especially come into action in the electricity sector. Almost 30% of the electricity consumed worldwide is already produced from renewable energy sources. But the electricity sector is only one part of the energy system. The energy transition has proved more difficult in the heating sector and especially in the transport sector. In the heating sector, which globally consumes about half of all energy, the share of renewable energy is about 10%. In the transport sector (excluding human-powered transport), which consumes about a third of all energy, the share of renewable energy is only 3–4%. Currently, renewable energy in transport consists mainly of so-called biofuels, i.e. liquid fuels processed from biomass, such as cereals or wood (and some biogas): the share of transport powered by electricity from renewable sources is much lower. However, the electrification of transport is one of the big trends in the energy sector.
FIGURE Renewable energy production by production mode 1965–2021. Today, hydropower still produces by far the largest share of renewable electricity, but in the near future, wind and solar power will overtake hydropower in popularity as a production mode.
Although the pace of change in electricity systems has been rapid, especially during the past decades, it is nowhere near sufficient to stay on the path to less than 1.5 degrees or, at least, less than 2 degrees of global warming.
So, what should happen?
Many pathways to energy transition have been outlined by governments, the EU and international organizations. The International Renewable Energy Agency (IRENA) believes that the electrification of societies based on renewable energy and energy efficiency are key to achieving the pathway of 1.5 degrees of warming. Given the very limited time available to carry out the energy transition to adequately mitigate climate change, pathway considerations must be based on options that are possible right now or in the near future. From a technical point of view, the tools for achieving the energy transition already exist and no currently unknown energy technology breakthroughs are necessarily needed. What is needed instead, is much more ambitious action than has been taken by governments and other actors so far (more on which in the fourth section of this course). Next, we take a look at energy transition based on IRENA's 2022 pathway analysis.
Massive and immediate increases in the production and direct use of electricity from renewable energy sources are needed
Both IRENA and the IPCC see increased renewable energy and more efficient use of energy as the cornerstones of the energy transition. A growing body of modellings shows that a carbon-neutral electricity system based on renewable energy is possible around the world. Limiting global warming to 1.5 degrees Celsius will require an unprecedented acceleration of renewable energy. By 2030, 65% of the electricity generated should come from renewable sources (26% in 2019), and by 2050 the share of renewables should be as high as 90% (see figure below). The biggest increase will come from wind and solar power. Hydropower (currently the largest source of renewable electricity), biomass, geothermal energy and tidal power will also play an important role in the transition. In IRENA's analysis, natural gas will still be used in 2050, but coal power will have been phased out. Increasing renewable energy capacity on this scale will not be possible without a significant strengthening of the electrical grids.
The figure shows how the electricity generation (the amount of electricity generated) and the electricity capacity (the combined nominal maximum capacity of power plants) would have to change by 2050 to limit global warming to 1.5 degrees Celsius. RE stands for renewable energy and VRE for weather-dependent renewable energy, i.e. wind and solar power. CSP means concentrated solar power, which uses mirrors to "concentrate" the sun's rays to produce electricity by evaporating water. In this scenario, as is typical of energy transition scenarios, electricity systems are expected to rely predominantly on solar, onshore wind and offshore wind power in 2050 (Source: IRENA 2022, 61).
The energy system will also have to adapt to the fact that in the future energy system the overwhelming share of energy will come from weather-dependent production (wind and solar). This will require the energy system to develop storage capacity and new levels of flexibility. Consumption must be much better adapted to the energy production available at the time in question. One example of the flexibility of the energy system is electric cars, which can be charged when electricity is plentiful and can offer their electricity storage for use when only little electricity is produced.
In addition to electricity production from renewable energy, the “direct” use of renewable energy must also increase significantly. This refers to the use of renewable energy in transport, heating and industry in non-electricity forms. In the past, direct use has been dominated by the use of “traditional” biomass (firewood and charcoal) for heating and cooking, but IRENA recommends that these should be phased out altogether due to fine particle emissions. One example of the direct use of renewable energy is solar thermal systems, where solar collectors are used to convert the sun's thermal radiation to heat domestic water and buildings. In heating, the use of geothermal energy, i.e. heat from deep within the earth, will increase. The use of biomass in transport, either as liquid fuel or biogas, will also increase. In IRENA's analysis of energy transition, on a pathway to less than 1.5 degrees of warming, biomass fuels will increase not only for road transport but also for shipping and aviation.
Such a transformation of the electricity system will, of course, require very large investments and policy measures to bring about change. IRENA estimates that the global annual investment needed to increase renewable energy will be approximately a trillion US dollars (USD) by 2050 (currently around USD 250 billion per year), and approximately USD 700 billion (currently around USD 275 billion) will be needed to strengthen the electrical grids and increase their resilience.
The barriers of the energy transition are not so much technological as political and cultural. A wide range of policy instruments to promote the transition of the electricity system have already been presented and tested. So far, the most effective way of achieving changes has been throughthe feed-in tariff scheme, which has also helped to kick-start wind power production in Finland. However, the use of available instruments requires political will.
Energy must be used more efficiently and less
In the energy use of buildings – where heating and cooling consume most of the energy – renewables will cover the majority of future energy needs, too. In addition, buildings will have to become more energy efficient, that is, the energy used per floor area will have to reduce significantly reduced. Energy renovations to reduce energy consumption have indeed become a key part of energy consumption regulation, for example in the EU. In IRENA's transformation pathway, all new buildings will be "zero-energy" buildings from 2030 onwards: they will consume no more energy than they produce themselves with renewable energy.
In the transition, the ways of heating buildings will move away from combustion-based energy production to energy-generating heat pumps that extract the energy needed for heating from air, water or ground. The electricity needed to run these systems would be produced from renewable energy sources, such as solar power produced by solar panels on the roofs of buildings. This transition can be achieved at all scales from small houses to apartment blocks. Additionally, district heating will be increasingly provided by heat pumps. According to IRENA, the investment needs for the energy transition of buildings are even bigger than those for the electricity system, as the transition is still in its early stages. IRENA estimates that investments of USD 2,285 billion per year will be needed between 2021 and 2030, and USD 1,185 billion per year between 2031 and 2050.
Final energy consumption in buildings worldwide in 2019 and in 2030 and 2050 according to the IRENA scenario. Globally, the floor area of buildings will increase, slowing down the reduction in energy consumption achieved through energy efficiency measures. In this scenario, buildings are calculated to become carbon neutral (Source IRENA 2022, 82).
The energy transition in the transport sector is a challenging one. Transforming transport to low emission, let alone carbon neutral, requires both technological and behavioral changes. As we learned in section 2.3.1, electricity appears to be winning the race for the future propulsion of road transport, although its share is still low and electricity is currently used far less than biofuels (around 1% vs. 3% of energy consumed in transport). In IRENA's 1.5 degree energy transition pathway, electrification of transport is key to achieving near zero GHG emissions by 2050. However, the scenario would also require a near five-fold increase in the use of liquid biofuels for transport over the same period.
This scenario would require electric cars to immediately become much more popular so that electric cars would account for the majority of cars sold globally in 2030 (8.3% in 2021). However, the growth of electric car sales will depend on the development of charging infrastructure, incentives to purchase electric cars and perhaps a ban on the sale of internal combustion engine cars. In addition, public transport and modes of human-powered transport should replace private cars, and not only short (human and freight) flights but also road freight transport should be shifted to electrified rail transport.
Energy consumption in transport worldwide in 2019 and in 2030 and 2050 according to the IRENA scenario (Source: IRENA 2022, 82)
Green hydrogen as part of the renewable energy system
An important part of the future energy system will probably be the use of hydrogen for energy transport, storage and production. The use of hydrogen is not a new invention; it has been known for centuries and over the last 50 years the enthusiasm for using clean hydrogen has grown. The oil crisis of the early 1970s was a shock to the energy system and sparked enthusiasm for hydrogen. As the oil crisis abated, so did the enthusiasm for developing hydrogen technologies.
Hydrogen itself is not an (unrefined) energy source, but must first be produced. Different hydrogen technologies are referred to by different color codes depending on the production method. Grey (or brown/black) hydrogen refers mainly to hydrogen produced from natural gas or coal, the production of which produces GHG emissions. Blue hydrogen is also hydrogen produced from fossil sources, but carbon capture and storage technology is used in the process, which means that most of the emissions from the process are removed. Green hydrogen is hydrogen produced from water using renewable energy.
Today, almost all of the hydrogen used is produced from fossil sources, but one of the very actively explored niches in the energy system is the production of green hydrogen. Hydrogen has a variety of uses – including non-energy uses – in heavy industry, and it is also used to a small extent in transport as a substitute for fossil fuels. In addition to the potential of these applications (see graph above for transport), one of the important uses of hydrogen in the energy transition will be to balance the electricity system as weather-dependent renewable energy production becomes mainstream. When wind and solar power are abundant, hydrogen can be separated from water for long-term storage, too, to be used later when wind and solar power are scarce.
The transition from a fossil economy to a hydrogen economy is not certain: as noted, enthusiasm has faded in the past, as well. Hydrogen has many obstacles to overcome. Because of its physical properties, it is not easy to store and transport and it requires a lot of energy to produce, and in addition, green hydrogen is currently expensive compared to grey and blue hydrogen. However, on IRENA's pathway to achieve the 1.5 degree target, 12% of global energy consumption would be covered by hydrogen in 2050.
Is there a place for nuclear power?
It should be noted that the energy pathway formulated by IRENA to limit global warming to 1.5 degrees is mainly built on technologies already on the market. No new energy technology breakthroughs are therefore needed to mitigate climate change. The IRENA analysis assumes that the share of nuclear power will remain at around 10% of electricity generation capacity.
According to the literature on energy transition, there is no place for large-scale nuclear power plants of the current scale beyond the lifetime of existing plants. On the other hand, there is considerable disagreement among researchers about the role that nuclear power solutions currently innovated will play. These include breeder reactors, small modular reactors (SMRs) and fusion power. None of these is exactly a new invention – some breeder reactors and SMRs have even been connected to the electrical grid. On the other hand, even optimistic estimates suggest that commercial deployment of fusion power is so far in the future that it will not be able to contribute to urgent climate action.
One potential addition to carbon-free energy production that is being actively discussed in Finland is small nuclear power plants. These are modular reactors (SMRs) that are much smaller (<300MW) than existing nuclear power plants. The components of small nuclear power plants could be (serially) manufactured in a factory and transported to the places of use. Similarly, when a part (module) of a power plant reaches the end of its useful life, only this part needs to be replaced by a new one.
No SMR solution has yet reached the commercial stage, but new power plant concepts are also under development. It is not yet known whether SMRs can be made sufficiently economically competitive on a significant scale. This depends not only on the future market price of electricity, but also on the evolution of the costs of alternative forms of generation, such as wind and solar power. As there are, of course, no examples of future commercial SMR plants, it is not yet known how policy guidance and regulation will affect their attractiveness as an investment. The public and political acceptability of small-scale nuclear power can only be seen once the plant processes are implemented. In any case, SMR plants will not be ready to help the energy transition, at least not during the 2020s.
The energy transition changes the geopolitical landscape
Since all modern societies depend on the constant availability of sufficiently cheap energy and, in practice, on cross-border trade in energy, energy has a very obvious geopolitical dimension. The changes in the geopolitical positions of different countries and regions as a result of the energy transition are one of the key issues in research on energy systems. It is a fairly common perception that the global political landscape will change radically and that a renewable energy system will cause less conflict than a fossil energy system.
The renewable energy system also poses geopolitical challenges. The growing use of renewable energy will benefit, on the one hand, countries and regions with well-developed industry and competence, such as the US, the EU and China, and, on the other hand, those with the necessary raw materials, such as China, Russia and many African and South American countries. The US, the EU and China, for example, are therefore beneficiaries of the energy transition. The economic pressure on countries that currently base their economies on fossil fuel exports, mainly OPEC countries in addition to Russia, could lead to international and national political instability.
Solar and wind power technologies also require the use of certain rare earth metals. There is a possibility of cartels developing around these materials, similar to the OPEC oil cartels that were behind the oil crises of the 1970s. China is the overwhelming market leader in the production of rare earth metals, which enhances its geopolitical position. Geopolitical tensions can be prevented by developing earth metal production chains also in countries other than China and Russia, by reducing the need to use rare earth metals through technological innovation, and by increasing the recycling of these materials. However, these measures may take a long time to implement, meaning that China's dominance is likely to continue in the future.
The threat of new “resource curses” is also linked to rare materials in countries where materials are abundant. Resource curse refers to the tendency of countries (or smaller communities) to focus and rigidly concentrate their exports on a resource that can easily generate economic benefits at the time, to the point where it saps the rest of the country's economic activity and production. This resource then becomes a major risk for the future development of the state: all eggs are in one basket, so to speak. For example, if market demand for this resource collapses or a competing country takes over the market, the national economy will take a huge hit, and this can lead to mutually reinforcing problems as public finances shrink, unemployment rises and the economy collapses. An example of resource curse in the last decade is Venezuela, a major oil producer whose economy went into free fall as oil prices fell. Resource curse is often accompanied by widespread corruption. On the other hand, the requirements for developing renewable energy are very different from those for oil, and more renewable energy can also lead to economic diversification.
In a world where renewables dominate the energy market, investment in renewable technologies and technology transfer, for example between developed and developing countries, can become a source of international cooperation but also of conflict. Conflicts can develop around renewable energy infrastructure, especially if new asymmetric interdependencies between countries emerge, making countries in dire need of energy or technology solutions easy targets for exploitation. The geopolitical implications of increasing cross-border electrical grids can also be manifold. Shared electrical grids can support cooperation between countries and thus also reduce the threat of conflict, but at the same time they increase, for example, the global vulnerability of electrical grids to cyber-attacks.
Would you like to collect something on this section? Voluntary.