1.2. Variables and indicators
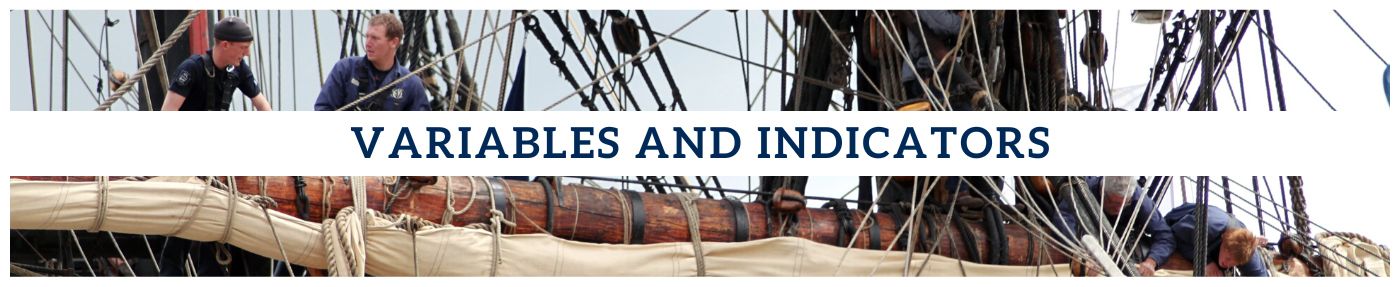
1.2 Variables and indicators
When it comes to sustainability, we know the key issues: climate change, biodiversity loss, and inequality between humans. On a global scale, inequalities are radical: some people do not have enough resources to meet even the basic needs for survival and health (nutrition, safe shelter, clean drinking water, sanitation, and basic health care), while others overconsume these resources (e.g., overconsumption of food, electricity and heating energy).
At the same time, therefore, there is a need to curb production and consumption to an ecologically sustainable level, but in certain regions and for certain groups of people, there is a need to strengthen the resource flows and structures that would enable meeting the needs. But how do we know whether a particular change in society and human systems will lead towards the objectives of the sustainability transition? Since change is slow and by no means always takes the shortest route to the desired outcome – for example, GHG emissions may decrease on one side and increase on the other – we need indicators and evaluation methods that allow us to compare the results obtained both between different actors and between different points in time. They would also help to assess the effectiveness of different instruments in contributing to sustainability objectives.
The most relevant indicators of the state of the Earth and human systems, as well as the indicators related to sustainability of development, have already been discussed in previous Planetary well-being courses. This course offers a brief review that focuses on the indicators that depict the current state of systems and help understand the scope of the change needed. In particular, we address these indicators from the perspective of sustainability transition: for example, what indicators are suitable for monitoring sustainability outcomes at different levels and what the strengths and weaknesses of different indicators are.
From a human-centered perspective, the overall state of a desirable and sustainable society has been described by the doughnut economy model made famous by Kate Raworth and since then widely applied (especially in research, still not so much in societies). Through ecological constraints and a minimum of social sustainability, the model defines a just and safe living space for humans, a “doughnut”, within whose limits a society can be said to be both ecologically and socially sustainable. The role of economic sustainability in the doughnut model is instrumental: it is a means to achieve the social and ecological objectives of sustainability, which are intrinsically valuable.
Using the doughnut model, a team of researchers led by Andrew Fanning conducted a global comparison of years 1992 and 2015 in 2022. The comparison shows how social development and the environmental impacts of human activities have changed over that time. As we learned in the Good life and planetary well-being course, the world is divided in regards to achieving ecological and social sustainability: countries have either managed to reach a proper level of social sustainability or have stayed within the limits of ecological sustainability but have not achieved both goals at the same time. The global picture, particularly when examined from an ecological perspective, provides crucial overall information on the direction of change, even though it obscures country-specific differences.
When it comes to social sustainability indicators, the world has made better progress at the global level since 1992 in areas such as access to energy, access to secondary education and perceived life satisfaction. However, the changes are relatively small, and at the same time, measured on ecological indicators, the situation has deteriorated relatively more, most alarmingly in terms of GHG emissions and resource use. Promoting social and environmental objectives simultaneously is thus very challenging, and there are often tensions and contradictions between these dimensions of sustainability, for example within energy and food systems (on energy, see e.g., Fuso Nerini et al. 2018).
The social and ecological state of the world pictured in doughnuts in 1992 and 2015 (Fanning et al. 2022.). The "ecological ceiling" of the graphs, that is, the upper edge of the doughnut, consists of four planetary boundaries (CO2 emissions or climate change, nitrogen and phosphorus cycle, terrestrial ecosystems or land use change, and freshwater use; see all planetary boundaries below), in addition to the ecological footprint and resource use. The ecological footprint measures the land area used by humans, both directly as a resource and as a sink for emissions. You can see the evolution of doughnuts in different countries here.
Food for thought: can you reember synergies, or maybe invent some?
Do you recall or can you imagine situations where the promotion of a particular social objective goes hand in hand with the accommodation of human activity within planetary boundaries (synergies)?
If you can't think of or recall any, you can go back to the Introduction to planetary well-being course (opens in a new window) and review the synergies discussion under the sustainable development goals tab in section 4 to identify synergies between social and ecological goals..
Ecological indicators: planetary boundaries
In the doughnut model, planetary boundaries are the ecological boundary conditions for a sustainable society: thresholds pertaining the planetary boundaries and indicating adverse environmental should not be exceeded. We have learned about planetary boundaries in previous planetary well-being courses. Will Steffen's research team has chosen the following as ecological variables for the planetary boundaries model (proposed indicator in brackets):
- Climate change (the level of carbon dioxide in the atmosphere, safety limit 350 ppm)
- Biodiversity integrity (no established indicator; rate of extinction has been proposed as an ad hoc indicator, as well as biodiversity intactness index)
- Ozone depletion in the upper atmosphere (the amount of ozone at a given point in the atmosphere)
- Ocean acidification (aragonite saturation, which determines how well some marine entities can form their calcium carbonate based structures)
- Nitrogen and phosphorus cycle (amount of nitrogen fixation in nature and industry; amount of phosphorus transported by rivers to the sea; amount of phosphorus transferred from fertilizers to soil)
- Land use change (area of remaining forest compared to the original forest cover)
- Freshwater use (global: consumption of water extracted from freshwater systems; regional: water extraction’s proportion of monthly average flow of rivers in the catchment area)
- Atmospheric fine particles (optical thickness of atmospheric aerosol AOD, safety limits are regional)
- Chemical pollutants and other new entities (these are still very poorly known, so no safety limit or indicator has been set)
Doughnut indicators as monitors of the sustainability of urban development
Indicators developed to monitor the situation at global level are often not very good at monitoring the situation at a more local level. Global indicators can be blind to local conditions and problems that require special attention at local level. In many cases, they are also too general to provide feedback on actions that cities, for example, can influence. However, the idea of the doughnut model and the planetary boundaries that form the ecological ceiling of the doughnut can be used to develop more local indicators. In Finland, for example, a team led by Tapio Reinikainen, which has been working on indicators for sustainable urban development, proposed the following indicators, in the spirit of the doughnut model, which are suitable for use at the city level, both in relation to social development and planetary boundaries.
Planetary boundary | Indicators of sustainable urban development |
---|---|
Climate change | Greenhouse gas emissions |
Renewable energy production | |
Share by transport mode (walking, cycling, private cars public transportation, other) | |
Biodiversity | The share of green areas in the urban environment of cities |
Area of nature conservation areas | |
Share of old-growth forests of total forest area | |
Air guality | Concentrations of fine particulate matter, nitrogen oxides (NOx) and sulphur dioxide (SO2) |
Population exposed to air pollution levels higher than WHO's new guideline values | |
Chemicals in the environmen | Perfluoroalkyl substances in raw water and wastewater |
Mercury levels in fish | |
Status of water bodies | Water purification percentages |
Nitrogen, phosphorus and BOD loads entering water bodies | |
Ecological status of rivers, lakes and coastal areas | |
Land use | Share of population and jobs in pedestrian and public transportation zones in cities and population centres |
New residential construction in pedestrian and public transportation zones | |
Natural resources and circular economy | Volume of municipal waste and recycling rate by waste fraction |
Land use efficiency | |
Material lows |
Development of human societies | Indicators of sustainable urban development |
---|---|
Equality, inclusion and opportunities to influence | GINI coefficien |
Urban segregatio | |
Accessibility of built environment | |
Accessibility of digital services | |
Voter turnout in different elections | |
Inclusion indicator | |
Health and mental health | Perceived wellbeing |
Positive mental health | |
Loneliness | |
Pupils who are bullied at school | |
Obesity |
|
Population morbidity index | |
Livelihood | Share of low-income households |
Unemployment rate | |
Homelessness | |
The share of housing costs (in disposable income) | |
Safety | Perceived safety |
Offences against Iife and health | |
Accidents | |
Education, research, development and business (level of activity) | Educational structure of the population |
Research and development expenditure |
|
Environmental goods and services sector | |
Sustainability of public finances | Employment rate |
Tax revenue | |
Debt/resident of public finance units | |
Urbanisation | Accessibility of recreational areas |
Accessibility of local services by public transportation or on foot | |
New construction and the expansion of built environment in urban and rural areas |
Indicators of sustainable development
The 17 main sustainable development goals (SDGs) of the 2030 Sustainable Development Agenda are well known, but at a more detailed level, the Agenda contains a total of 169 sub-goals (https://www.stat.fi/tup/kestavan-kehityksen-yk-indikaattorit-agenda2030-kuviot-ja-taulukot_en.html), with 231 indicators that measure their success (https://unstats.un.org/sdgs/indicators/indicators-list/). If you wish, you can learn more about how the goals were created under the Sustainable development goals tab in section 4 of the Introduction to planetary well-being course. You can follow the state of the world and its evolution regarding the 2030 Agenda goals on the open database https://unstats.un.org/sdgs/dataportal.
In addition, for example, the OECD publishes "Measuring Distance to the SDG Targets" reports, which describe the progress of OECD countries (in practice, high- and middle-income countries) towards the 2030 Agenda targets. According to the latest report, published in autumn 2022, OECD countries as a whole have achieved or nearly achieved 28 of those 112 Agenda 2030 targets that are considered possible to measure in terms of change.
Example visualization: how are OECD countries progressing towards the 2030 Agenda targets? Full graph and more details (p. 3).
The focus of the sustainability transition differs from the SDGs in that the ultimate goal of the sustainability transition is to achieve the objectives of ecological sustainability. This is because most of the Agenda 2030 goals will not matter and cannot be achieved or safeguarded unless the underlying threats to human well-being and societal stability are addressed, that is, if climate change won’t be mitigated and the biodiversity loss halted. Climate change and biodiversity loss are linked to processes at the Earth and ecosystem level, where there is an obvious risk of feedbacks that reinforce adverse trends if action to reduce the negative impacts is not sufficiently effective. Therefore, the sustainability transition underlines the urgency and scale of environmental actions.
The relative weighting of the 2030 Agenda goals must be considered differently in different societies. In low-income countries, the task is above all to promote equal opportunities for well-being and achieve social sustainability (in ecologically sustainable ways), while in high-income countries (and increasingly in middle-income countries) the task is sustainability transition, i.e., the alteration of human systems and their processes and the transformation of lifestyles to fit within the limits of ecological sustainability (in socially sustainable ways). As such, the Agenda 2030 set of goals and indicators may provide a meaningful overall framework for sustainability work at local level, for example, where the SDG is broken down into more concrete sub-targets, such as the MayorsIndicators tool that is designed to assist municipal sustainability work (https://www.mayorsindicators.com/) or the “doughnut indicators” for sustainable urban development presented above.
Variables of planetary well-being
Planetary well-being emphasizes the functional integrity of Earth and ecosystem level processes. It is the basis for both human and non-human well-being. Planetary well-being fits well into the sustainability transition thinking, as it emphasizes the fundamental importance of protecting the aforementioned processes.
What distinguishes planetary well-being from the sustainability frameworks presented earlier is that its definition of sustainability is based, on the one hand, on processes and interactions and, on the other hand, on the non-anthropocentric values that we discussed in the Good life and planetary well-being course. For the planetary well-being sustainability value set, it is not enough to protect
people's opportunities to pursue well-being: we must also protect the opportunities for other forms of life to pursue well-being.
Many previously developed indicators can be used to support the pursuit of planetary well-being. The overall global state of planetary well-being is fairly well captured by the Red List Index (RLI), an indicator of changes in the state of biodiversity. The RLI is a kind of "thermometer" of planetary well-being: if processes important for well-being are disrupted, this is reflected in an increasing number of species that become threatened or endangered. The situation described by the Red List Index and the Red List (https://www.iucnredlist.org/) shows that we are far from planetary well-being. However, the symptom index does not tell us what is actually causing the situation, and there is a considerable delay before the index shows the changes that have been done to correct the situation. It is therefore important from planetary well-being’s perspective to look for indicators that can be used to assess the state of processes and related systems that are important for well-being.
The planetary boundaries discussed earlier concern the states of processes and systems that are also essential for planetary well-being. They provide interesting indicators, but their “scales” need to be adjusted to the needs of planetary well-being, as the current safety limits are defined on the basis of an anthropocentric value system. For example, in the light of current knowledge provided by IPCC, a global warming of 1.5 degrees Celsius would help to avoid dangerous climate change from a human perspective. However, a world 1.5 degrees warmer (especially at the rate climate change currently progresses) is damaging or dangerous for many other ecosystems and their species. For example, coral reefs and the species depending on them are suffering significantly already – let alone as warming intensifies. On the other hand, any action that mitigates climate change and the rate of its progression will also significantly reduce the harm to species and ecosystems particularly vulnerable to climate change compared to the current situation.
So far, the research community has not come up with any separate indicators of planetary well-being. However, in terms of the potential for well-being of all living things, certain measurable processes already presented in the planetary boundary model emerge naturally as suitable candidates: for example, climate change (global carbon cycle processes) and biodiversity loss (which directly reflects the unrealization of planetary well-being in an extreme and irreversible way) are key variables for planetary well-being. Similarly, for example, ocean acidification causes serious disruptions to processes that are essential for the well-being of marine ecosystems and, indirectly (e.g., through food chains), of many species in other ecosystems.
The choice of the indicator is one thing, the target value set for it is another. A sustainability transition towards planetary well-being would require more ambitious goals, for example for climate change and biodiversity loss. Safeguarding the conditions for the well-being of all living things would require tighter restrictions on global warming and slowing down climate change. In terms of biodiversity loss, virtually all human-induced species loss and extinction, whether direct or indirect, is a manifestation of activities that undermine planetary well-being.
On the other hand, the idea of planetary well-being, that emphasizes the functioning of processes at Earth and ecosystem level, does not properly answer the question of how to assess the impact of spatially limited human activity on these processes and what, in that case, would be the limit set on the sustainability of actions. For example, local land use can be so spatially limited that it does not yet have a decisive impact on the well-being, let alone survival, of any particular species- But if similar choices are made in all neighboring municipalities, resulting in, for example, a significant shrinkage or disappearance of a large forest area, the well-being of certain species or at least certain populations will be decisively reduced.
Similarly, it is not clear, for example, what would be a sustainable level of GHG emissions in a city that produces steel or concrete to meet the needs and enable the well-being of its own people and those of neighboring countries. Similar examples can be found for all types of human activity that are related to meeting human needs but whose production is concentrated on a certain region (including food production). Measuring sustainability at different scales is therefore an important topic on which the research community is actively generating new knowledge.
Absolute and relative indicators
Using and reading indicators requires an understanding of how scales and differences between reporting relative and absolute results affect the results of the measuring. Take, for example, the measuring of activities that enhance or mitigate climate change. Absolute measures include carbon dioxide concentration in atmosphere and global anthropogenic GHG emissions. Relative measures include, for example, GHG emissions per person or per euro compared to a country's economic activity (GDP). For example, climate action can be expressed in terms of absolute or relative emission pledges. A pledge to reduce emissions per unit of economic activity (CO2-eq / € GDP) is an example of an indicator and pledge linked to relative emissions. However, such an indicator can also present an activity in which total emissions are increasing as sustainable if the economy is growing rapidly at the same time. The danger of greenwashing is therefore obvious when using certain relative indicators.
Moreover, using the value of an activity’s resource efficiency as a measure of sustainability is overshadowed by the so-called Jevons paradox: when the efficiency of using a resource increases, it often also leads to a significant (at worst, relatively equally burdensome) increase in consumption around either the same or another activity, with no real absolute savings. The economist William Stanley Jevons drew attention to this as early as 1865 in his book The Coal Question: despite the enormous improvements in the fuel efficiency of steam engines, the amount of coal burned in England had increased by about 100 times during the 19th century.
An everyday modern example: although the energy efficiency of many household appliances has improved significantly over the decades (the A–E rating of energy efficiency is becoming obsolete, with many new products in many appliance categories all being in the top A group), the number of electrical appliances we use and the volumes of use have increased so enormously that the total annual household electricity consumption in Finland has risen from around 5 terawatts in the 1970s to over 20 terawatts (TW). Similarly, the total energy consumption (in terajoules, TJ) in Finnish transport has remained stable or even increased slightly since the 1990s, despite a significant drop in fuel consumption per kilometer of cars (https://stat.fi/en/statistics/ehk).
For absolute indicators, the scale of monitoring can be misleading. Monitoring the sustainability of an activity using only an absolute indicator may create an illusion of sustainability if the actor is of small size. For example, the lifestyle of a small nation would always appear to be ecologically sustainable if the indicator is the emissions or resource consumption produced by the nation as a whole, which is of course marginal compared to global emissions. When comparing and assessing the environmental sustainability of countries, for example, it is therefore important to set the emissions in proportion to population and to take into account the emissions that are outsourced to the rest of the world, i.e., the ecological impact of imported goods and food. This is the only way to ensure that a country does not carry out a false sustainability transition by outsourcing its production.
Both absolute and relative indicators are necessary, as they can be used for different purposes. However, one must be careful with the use and appropriateness of the indicators and with the conclusions drawn from the data produced by certain indicators.
Would you like to comment something on this section? Voluntary.